WORKING GROUP REPORTS
Major Shifts in Species Composition and Ecosystem Structure
Cochairs: A. MacCall and P. Kremer
Participants: W. Graham, R. Haney, A. Hollowed, D. Mackas, M. Ohman, T. Powell, G. Rau, J.
Rice, J. Schumacher, L. Shapiro, P. Smith, L. Welling, and E. Woehler
PROBLEM DEFINITION
Time series of physical and biological measurements in eastern boundary currents (if not in the oceans
in general) exhibit nonstationary properties: abrupt changes in descriptive parameters such as mean,
variance, or phase relationships that are seemingly unpredictable and inconsistent with those from a
preceding time period. Isaacs (1976), who referred to qualitative states as "regimes," described this
problem as follows: "There are internal, interactive episodes locked into persistence, and one is
entirely fooled if one takes one of these short intervals of a decade or so and decides there is some sort
of simple probability associated with it....fluctuations of populations must be related to these very
large alternations of conditions."
Qualitative shifts in physical properties, species composition, and ecosystem structure may exist on a
variety of spatial and temporal scales, but in the California Current they are most obvious in long-term
records of temperature and fish abundance. The historical temperature record from the Scripps Pier
shows three prolonged periods of different mean annual temperature (Fig. 10). The middle period,
extending from the early 1940s to the mid 1970s, is so much colder than the adjacent warm periods
that the warmest years during the middle period (with the exception of the 1958-59 El Niño) are all
below the average temperature of the warmer periods (MacCall and Prager 1988). Although the
Scripps Pier record is local, SST observations from ships show the recent warming to encompass the
entire northeastern Pacific rim, from the equator to the Aleutian Islands (Fig. 11). Again, the lack of
cold anomalies since 1976 is striking. From an ecosystem perspective, temperature is only an easily
measured proxy variable, and is related to a suite of physical conditions that in turn influence
biological processes. Major biological shifts in the California Current ecosystem have been
documented for the recent warm period, including a drop in zooplankton abundance and vigorous
recovery of the previously depleted Pacific sardine (Barnes et al. 1992).
Despite our recognition that time series of physical and biological oceanographic variables often exhibit
nonstationary properties, this concept is overlooked in actual practice. In many cases, nonstationary or
qualitative shifts are ignored in order to simplify models to a tractable level. This is especially the case
where spatial and temporal coverage are limited. Ambitious large-scale observation and modeling
programs such as the World Ocean Circulation Experiment (WOCE) are necessarily still at the level of
describing a single pattern of ocean circulation. Similarly, applied models of biological productivity,
such as fishery management and marine mammal population models, are typically based on
simplifying assumptions of constant reference points such as equilibrium-unexploited abundance.
RELEVANCE TO THE CALIFORNIA CURRENT SYSTEM
The number of historically observed transitions is small, but it is reasonable to infer that qualitative
shifts in state are typical of the California Current system and that they will continue to occur every
few decades. Qualitative shifts in this and other eastern boundary currents pose a suite of challenging
problems (Lluch-Belda et al. 1989).
SYSTEM UNDERSTANDING
Although the California Current is one of the best-studied regions of the world's oceans, our present
understanding of its physical and biological oceanography is inadequate to explain or predict the
qualitative shifts we have observed. With the exception of El Niño, these shifts have been overlooked,
probably because studies have not included appropriate time and space scales to approach the problem
quantitatively. Recent developments in the mathematics of dynamical systems (e.g., "chaos")have
demonstrated that qualitative-state shifts can arise from rather simple nonlinear models (e.g., May
1986). With this realization, augmented by a new kit of conceptual "tools", the problem of qualitative
shifts is now emerging as a legitimate and fundamentally important area of oceanographic
investigation.
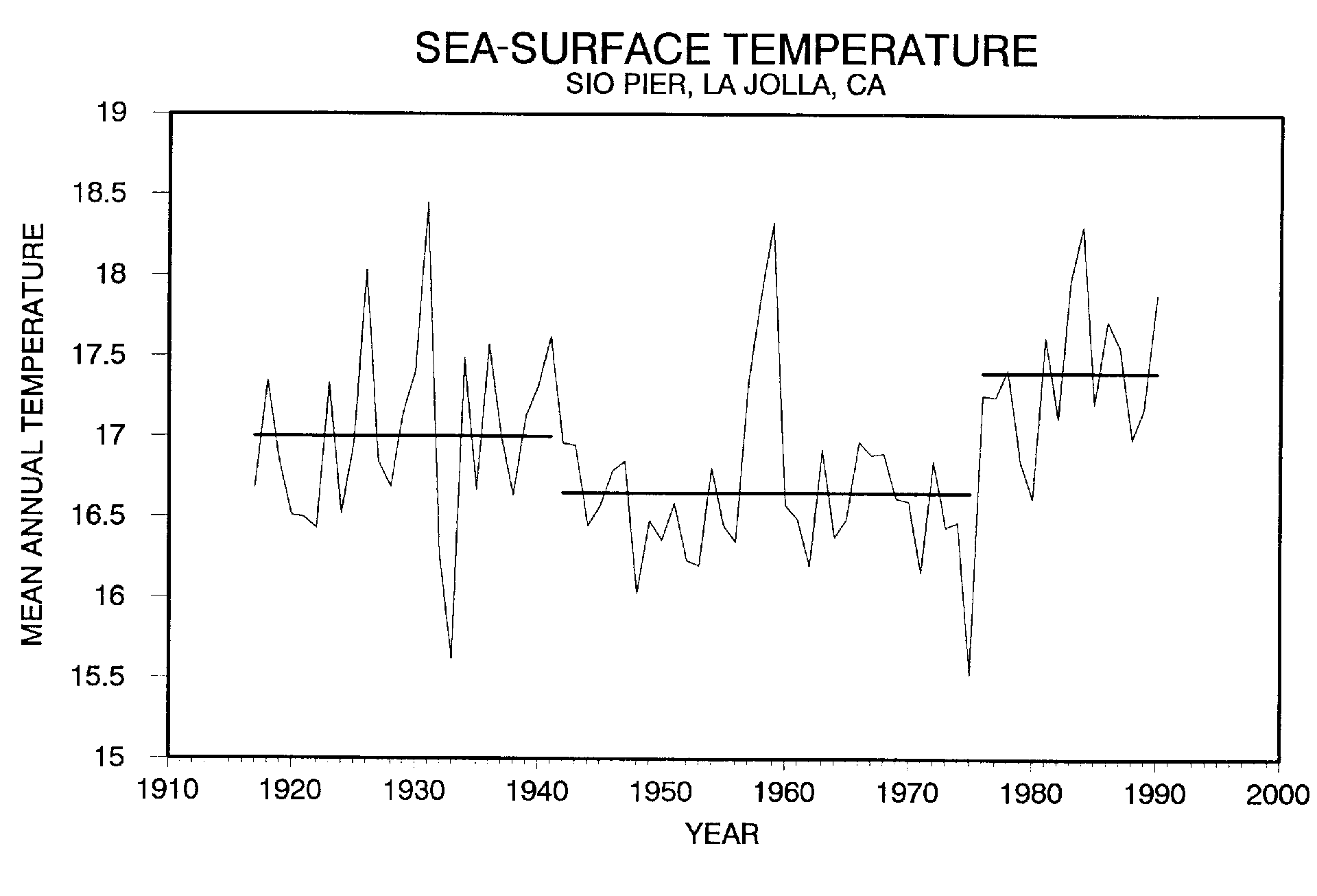
Figure 10. Mean annual sea-surface temperatures observed at
Scripps Pier, La Jolla, California. Long-term averages are shown for
three qualitatively different periods.
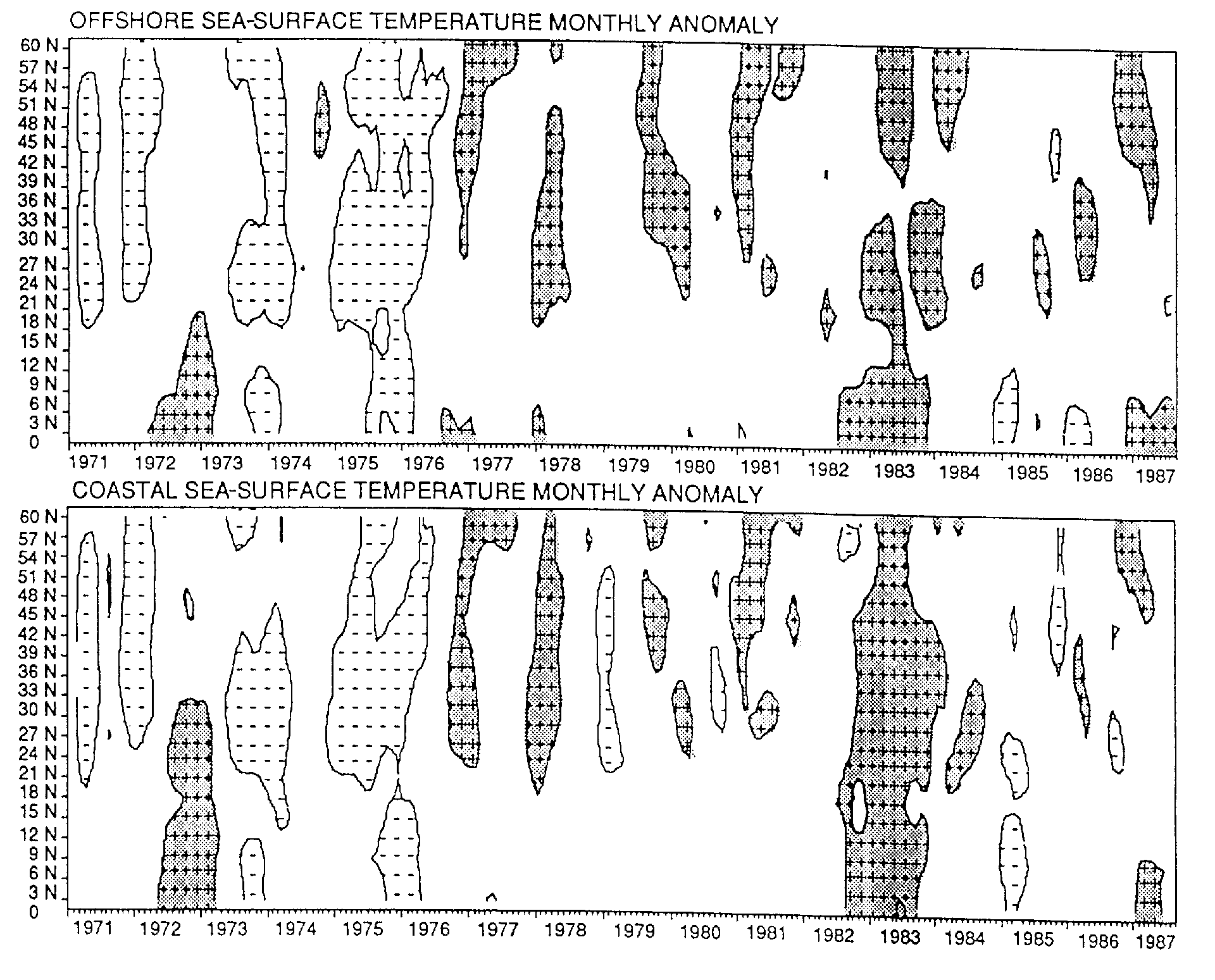
Figure 11. Anomaly of monthly mean sea-surface temperatures from
ships of opportunity (Cole and McLain 1989). Contours are (+/-) 0.5
degrees Celsius, positive anomalies are shaded. "Coastal" is
approximately 0 to 200 km from shore; "offshore" is approximately 200
to 600 km from shore.
Although qualitative shifts are most noticeable and best documented at large scales in space and
time, there is a spectrum of conceptually related phenomena ranging in size down to much smaller,
localized scales. For example, the zooplankton in one parcel of water may be dominated by
crustaceans (e.g., copepods and euphausiids) while zooplankton in a nearby parcel may primarily
comprise pelagic tunicates (salps and doliolids) . Physical circulation patterns have been documented
to be important in determining the spatial distribution of the zooplankton, but we lack appropriate time
series measurements to determine the mechanisms that cause qualitative difference in the zooplankton
community. Zooplankton species compositions and abundances directly affect fisheries recruitment.
The processes leading to localized alternative states may provide insights into mechanisms that are
important at longer and larger scales. Although these short-term localized changes are more amenable
to direct study than long-term ecosystem shifts, short-term studies should not be viewed as substitutes
for long-term investigations. Research on state shifts must necessarily cover the full range of time and
space scales.
RESOURCE MANAGEMENT
Resource management policies that incorporate qualitative shifts in ecosystems have not yet been
developed. Models commonly used for managing living marine resources assume a steady state,
perhaps with allowance for environmental "noise." On the west coast of North America, this steady
state assumption is clearly inappropriate even in the absence of global climate change: qualitative shifts
in species composition and structure appear to be a property of the ecosystem. Many resources and
industries may be at risk if qualitative ecosystem shifts result in inappropriate management
expectations and responses. Eastern boundary currents are known for their spectacular fishery
collapses such as those for the Monterey sardine and the Peruvian anchoveta. Such collapses seem to
be an inevitable consequence of inadequate understanding of the resources and the ecosystems. The
required knowledge consists of (1) improved resource management models based on understanding of
qualitative-state shifts; and (2) improved capability to predict state shifts or to recognize them as early
as possible after they have occurred. It is doubtful that adverse fluctuations in the stocks and related
industries can be avoided, but if management were armed with the above knowledge and acted
appropriately, it should be possible to reduce the severity and duration of the downturns and their
resultant economic and social hardships.
GLOBAL CHANGE
Qualitative shifts in ecosystem state occur normally. Although there is no assurance that past states will
recur under conditions of global warming, past ecosystem behavior is still our best source of clues
about future states and dynamics. Logical induction leads us to the hypothesis that, at least in the
California Current system, physical and ecosystem response to global change (whether or not the
change is anthropogenically forced) may consist of abrupt changes in qualitative states (e.g., a step
function) rather than the gradual change suggested by the smooth forcing function of increasing
atmospheric greenhouse gases.
This possibility has profound implications not only for timely detection of the effects of global climate
change, but also for planning appropriate societal responses. Therefore, investigations of the response
of eastern boundary currents to global climate change must be designed to encompass these changes.
We have referred to state shifts as "qualitative," because of their most noticeable properties, but it is
nonetheless essential to describe and understand these phenomena quantitatively. GLOBEC-sponsored
research on qualitative shifts will provide the understanding necessary to fully consider the effects of
global climate change on the California Current system and similar eastern boundary currents.
KEY RESEARCH QUESTIONS
- What controls the shift from one qualitative state to another? To what extent are qualitative shifts
predictable? What are the time and space scales of these shifts and their transitions? What ecosystem
properties are conserved across state shifts? Are the mechanisms initiating the shift different from
those maintaining the state within a qualitative regime?
- What are the relationships and linkages between physics and biology in causing and maintaining
qualitative shifts? Do changed physical conditions force a specific ecological response, or do they
allow a suite of possible responses? What generates hemispheric-scale shifts in physical and biological
systems? How do seemingly small differences in properties or histories of water masses result in large
differences in the biological communities inhabiting them? What is the relationship between changes in
space and changes over time? How can we better distinguish between advective processes and in situ
dynamics?
- What is the significance of initial conditions? Are the characteristics of qualitative shifts determined
by initial conditions, or is the end state independent of those conditions? What is the importance of "
seed " populations?
- What are comparative life-history strategies of species or populations impacted by qualitative shifts?
Do endemic species respond differently from more widespread species? Are there large-scale trophic
consequences to local shifts in invertebrate populations?
- At what point in time (or space) are qualitative shifts identifiable, and how much change is required
to constitute a shift? Are there characteristic physical or biological precursors? Are there convenient
proxy variables for monitoring system status and early detection of shifts? What are appropriate
management strategies for harvested or conserved living resources - strategies that recognize the
frequency and impact of qualitative shifts? What are appropriate management (and research) responses
if a shift is believed to be imminent?
PROPOSED PROGRAM: EXAMINATION OF HISTORICAL AND PALEOSEDIMENTARY
DATA
Long time series of physical and biological observations exist from a number of sources for the
California Current system (CCS). CalCOFI samples of fish larvae and larger zooplankton constitute a
detailed 40-year time series (1951-91) for a major portion of the CCS. Catch records of commercially
important fish have been kept for 75 years. Paleosedimentary records from anoxic basins provide data
essential for documenting qualitative shifts on the time scale of decades and longer (see Section 3.3).
In addition to directly examining material preserved in sediments, we need to identify proxy variables
that reliably indicate physical and biological conditions.
Analyses of historical data will provide the basis for identifying shifts in ecosystem structure and
suggest hypotheses for mechanisms or processes that may govern these shifts. These hypotheses can
be tested within the context of the modeling and field effort or by comparing results with historical data
not used previously to generate the hypotheses (e.g., cross-validation). They can also be validated or
invalidated by future monitoring efforts.
MODELING APPROACHES
The working group advocates two complementary, converging lines of modeling: process-oriented
models and process-neutral models. Process-oriented models are particularly appropriate where
processes are relatively well understood, as in physical oceanography. However, effects of some
relatively well-known physical processes such as wind-induced turbulence may be better modeled by a
"process-neutral" transfer function (wind speed cubed), which concisely describes the results of the
process without explicitly modeling the process itself (surface wave dynamics, etc.). When
less-well-understood processes must be included in a larger model, process-neutral models may be
required. These may be drawn from a large family of models including probabilistic models (e.g.,
Markov models) and empirical transfer functions that may be nonlinear and incorporate appropriate
time delays.
These process-neutral models form a natural beginning point for the evolution of more specific models
tailored to the processes and mechanisms of the California Current. Process-oriented models follow
naturally from neutral models as more information is gained . The process-oriented model then
becomes available to replace the process-neutral model, depending on the modeling context. Ideally,
interaction between construction and analysis of models and conduct of field research strengthens
investigations in both areas.
PHYSICAL OCEANOGRAPHY
Ecosystem shifts appear to be closely associated with changes in physical conditions. Better
knowledge of the physical processes and characteristics of alternative system states is needed in the
field of physical oceanography. Such knowledge would clearly help to explain qualitative shifts in the
biology of the system. Improved circulation models (including patterns and effects of upwelling,
advection, and transport) are needed. Beyond describing "average" conditions (the significance of
which becomes questionable in view of nonstationarity and discontinuous qualitative shifts), we need
descriptions of alternative physical states, and knowledge of mechanisms or processes that generate
shifts between (and persistence within) those alternative states.
BIOLOGICAL MODELS
Biological systems are laden with many properties that applied mathematics has shown to generate
complicated temporal and spatial behavior, and the phenomenon of qualitative biological shifts is a
natural consequence. Some examples of these properties are nonlinear responses to physical and
biological changes of the sorts often encountered in the recently developed field of "dynamical
systems"; plasticity in trophic relationships among species (especially given individual development
from larva through adult, spanning numerous trophic levels); effects of time lags; and continuous
spatial (re)partitioning of populations. A mix of process-oriented and process-neutral models is
necessary, and that mix will evolve with improved understanding.
Stability properties of ecosystems may arise from specific processes or mechanisms, but alternatively
could arise from more general properties of the component physics, organisms, and ecological
linkages. In the latter case, process-neutral models may guide subsequent research in several ways,
including identification of dependencies that are likely to constrain system trajectories or maintain
alternative states; identification of stable and unstable assemblages or configurations of the ecosystem;
and determination of model sensitivity to assumed structure or parameter values.
FIELD STUDIES
Initially, field studies will concentrate on mechanisms governing qualitative-state shifts in systems
small enough that transitions can be observed. This research would concentrate on many of the key
questions listed above (e.g., predictability of qualitative shifts, comparative life histories of species,
physical and biological precursors). The study of long-term qualitative shifts must rely on historical
records, archived samples, and proxy or indicator variables. Efforts to model qualitative-state shifts
will identify numerous features and processes requiring clarification and better understanding through
field study, including identification of critical mechanisms or sensitive leverage points, and estimation
of parameter values for use in models of key processes. Identification of specific areas of study is
premature, but an ambitious GLOBEC field program is likely to result. Increased field activity should
be anticipated as the program develops, and funding should vary accordingly.
PRODUCTS
Understanding the mechanisms and causes of long-term qualitative shifts in the California Current
ecosystem poses a major intellectual challenge. If we are successful, our knowledge will provide a
basis for monitoring and forecasting that would clearly benefit society. Even if prediction proves
unfeasible, earlier recognition of qualitative shifts would also be beneficial. Therefore, a major product
of this proposed GLOBEC research program is the development of a physical and biological
diagnostic capability that could be implemented by an agency such as NOAA.
The proposed prediction and detection capability would be supported by a low-cost monitoring
program. Design of that program will follow the research conducted under this GLOBEC program,
but some aspects can be anticipated: presumably, existing climatological observation sets (e.g.,
information from ships of opportunity and coastal stations, atmospheric pressure fields) would be
emphasized. A variety of satellite-based sensors may be expected to provide synoptic views of some
variables of the coastal ecosystem. These could be supplemented by low-cost opportunistic biological
samplers such as Hardy Continuous Plankton Recorders to obtain detailed information about shifts in
species composition and distribution of zooplankton, with emphasis on possible indicator species.
Other indicators of shifts (recruitment strengths, growth rates, physiological traits) may be extracted
from routine biological monitoring of commercial fisheries at little incremental cost to ongoing
sampling programs.
The combined monitoring and analysis could produce indexes of environmental indicators analogous
to those published by the Department of Commerce for the U.S. economy. NOAA has already moved
in this direction by initiating an annual compendium of environmental indicators. The program
described here differs from the NOAA effort in two important ways. First, the indexes would be
restricted to a better-defined system: the California Current off the west coast of the United States and
Canada. Second, the indexes would be better focused, having been developed and selected on the
basis of mechanisms and relationships identified by the research program.
The California Current is an ideal laboratory for developing such a predictive system. The background
of knowledge and historical observations is among the best in the world, and provides a solid base
from which to work. Successful effort in this system will point the way for similar programs in other
coastal and oceanic systems.